Sea / Offshore
NBS approaches enhance water quality, regulate nutrient levels, and support the overall health of open sea ecosystems. The following examples demonstrate just a few ways these solutions can address issues such as oil spills, plastic pollution, eutrophication, and harmful algae blooms (HABs).
Artificial Islands
Natural attenuation refers to the breakdown of pollutants in the environment through a combination of biotic and abiotic elements, including substrates and aquatic mediums, along with associated processes. An illustration of this concept is seen in the development of the Marker Wadden (2016-present), a newly established wetland within the expansive Markermeer, one of the largest lakes in the Netherlands (Figure 5-7) (Deltares, 2016) .
The establishment of the Marker Wadden serves a dual purpose: mitigating flood risks and expanding agricultural land by creating polders. This initiative traces back to 1923 when the Afsluitdijk was constructed, effectively isolating the North Sea and forming the Ijsselmeer. Subsequently, the “Houtrib” or Markerwaarddijk was built in 1976 to divide the lake into two distinct parts—the brackish Ijsselmeer to the north and the freshwater Markermeer to the south.
Despite the initial intention of creating polders, the polder plan was eventually abandoned, resulting in two large bodies of water with unnatural hydrology and limited biodiversity. This shift led to the accumulation of substantial amounts of fine sediment due to the severed connection to the sea, thereby disrupting the ecosystem.
Decades later, RHDHV participated in the NMIJ program ('Natuurlijker Markermeer IJmeer'), collaborating with the government to devise strategies for establishing a sustainable and resilient ecological environment. As part of this initiative, a notable field experiment was conducted, involving the formation of a wetland area constructed from the same soft sediments (mud) that had been dredged from the original lakebed. This endeavor demonstrated the feasibility of constructing wetlands and islands using such sediment. Eventually it was colonised by vegetation, becoming a breeding ground for birds and other wildlife, improving water quality, creating a new area for recreation, and other co-benefits such as CO2 sequestration (Royal HaskoningDHV, 2022).
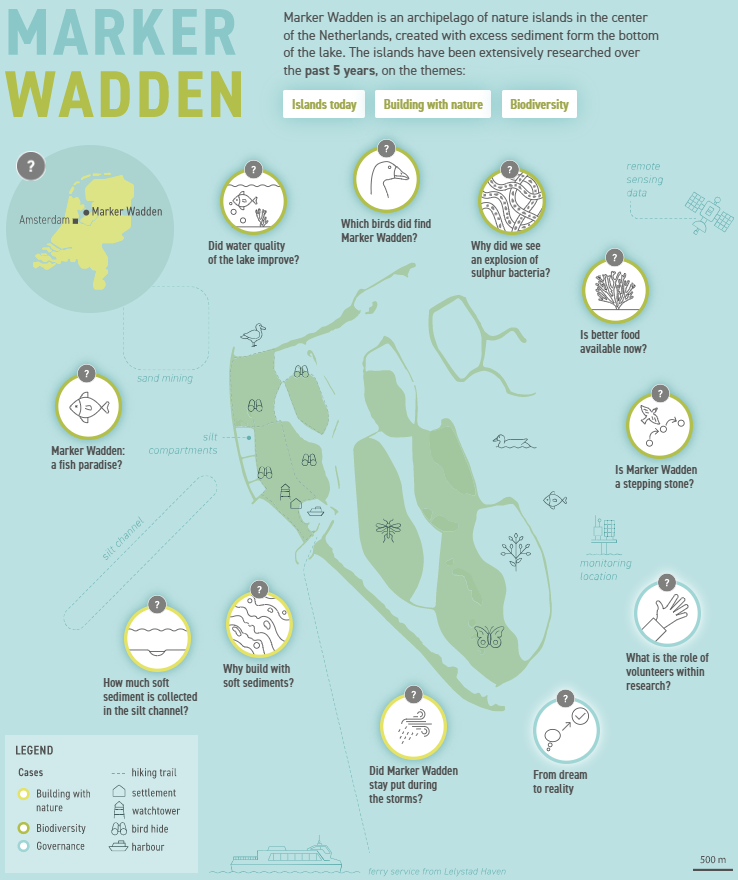
Table 5‑7: Project specifications for Marker Wadden
Indicator | Specification | Description |
Location | Place | Marker Wadden, The Netherlands |
Date | Project phase | 2016 – 2023 (and ongoing) |
Type of NBS | Constructing artificial islands with in-situ dredging | |
Treatment type | Deepening the lake with dredging to create clearer water. Building islands with the dredged material to allow for natural habitat creation, oxygenation, more currents, mixing, and natural attenuation of pollution. | |
Costs | Construction | €75 million first phase |
Operation (cost / year) | n/a (not applicable) | |
Scale | Gross | ̴ 1300 ha |
Partners and financial mechanisms | Design | Consists of 7 islands mostly for biodiversity restoration and water quality improvements, partly for recreation and tourism |
Realisation | co-financed: €15M Dreamfunds (National Postcode Lottery), €30M (Dutch Government), €3.5M (Province Flevoland), remaining from province North Holland, EU and private parties – including RHDHV for technical assistance. |
Natural Containment and Remediation of Oil Slicks with Microbes
Conventionally, oil spill clean-up involves the use of polypropylene booms and barriers, along with methods like oil skimmers, fire, and chemical dispersants, despite their environmental hazards. A nature-based alternative involves utilizing hydrophobic and biosorbent materials like hair, which repels water and collects contaminants such as oil. This approach taps into the renewable resource of abundant hair. A University of Technology Sydney study found that dog fur and human hair were effective for oil spill clean-up. The concept involves repurposing hair clippings into absorbent hair mats, as depicted in Figure 5-8, to capture oil and purify water. These mats can absorb up to five times their weight in oil. In 2001, Phil McCrory and Matter of Trust initiated the Clean Wave program, gathering hair, fur, wool, and fleece clippings through donations to create petroleum spill clean-up booms. This effort, combined with citizen science and volunteer initiatives, has significantly reduced major ocean oil spills. Although large-scale spills have decreased, everyday sources like car oil contribute to water pollution. Hair mats find application in storm drains, wells, filtration systems, rivers, urban areas, and airports. Sausage-shaped hair booms act as protective barriers along shorelines, preventing soil erosion and safeguarding natural ecosystems.
Certain techniques such as low-pressure flushing and vacuum pumping can disrupt and cause long term damage to sensitive microbial environments such as marshes and wetlands (Safina, 2011). Following the BP oil spill (Deepwater Horizon in 2010), traditional cleanup methods like low-pressure flushing and vacuum pumping were found inadequate and damaging to sensitive environments like marshes and wetlands. An alternative, more sustainable approach emerged, utilizing microbes for oil cleanup, which proved effective and cost-efficient. Bioremediation techniques, including bioaugmentation and biostimulation, were extensively employed. Bioaugmentation involves introducing oil-degrading microbes into affected areas to enhance degradation, while biostimulation stimulates the growth of indigenous oil-degrading microbes by providing essential nutrients. These methods were successful during the BP oil spill crisis, offering longer-lasting remediation effects. Researchers also identified A. borkumensis, a petroleum-consuming bacterium, as a potential asset for oil spill remediation due to its ability to thrive on hydrocarbons.
Additionally, biostimulation involves adding nutrients like nitrogen and sulfate to stimulate the growth of indigenous oil-degrading microbes, thus accelerating the rate of remediation. This method is preferable when native microbial communities are capable of efficiently breaking down oil. In high hydrocarbon environments, microbes convert them into biomass, needing nitrogen, phosphorus, and sulfate for growth (Microbewiki, 2022). Nitrate and sulfate fertilizers were added during the BP spill to promote oil-degrading microbes. Despite these nutrients in the Black Sea, they may not be the primary limitation for biostimulation, as bilge dumping sites intersect with algal blooms. Nonetheless, ongoing research continues to explore innovative bioremediation strategies for future oil spill events.
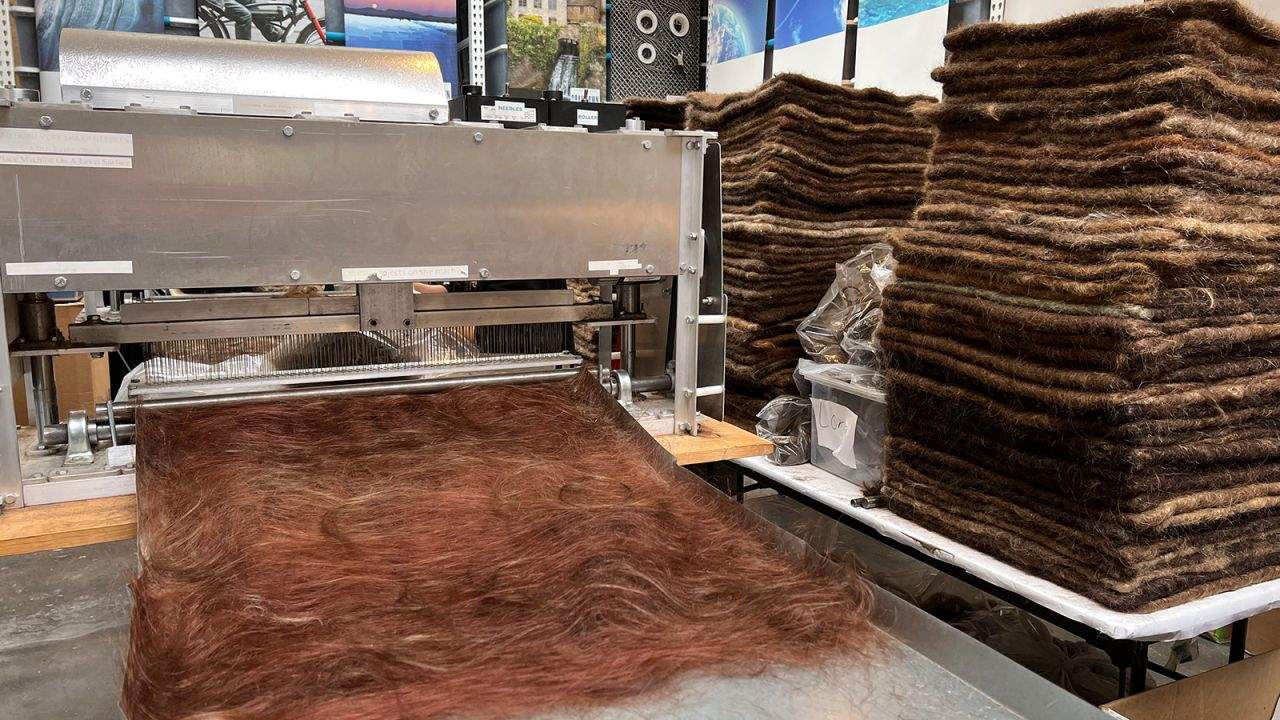
Table 5‑8: Project specifications for oil slick natural remediation with microbes and containment
Indicator | Specification | Description |
Location | Place | Oil Slicks, Gulf of Mexico, Deepwater Horizon (BP) |
Date | Project phase | 2010 and ongoing |
Type of NBS | Bioremediation (bacteria), adsorption (hair) | |
Treatment type | Containment through adsorption and anabolization through bacteria | |
Costs | Construction | Labour costs (hair is donated), bacteria is cultured and innoculated into spill area |
Operation (cost / year) | n/a | |
Scale | Gross | ̴ 6,500 to 176,100 km² (SkyTruth, 2010) |
Partners and financial mechanisms | Design | n/a |
Realisation | ~ $70 billion over 10 years is the compensation cost to BP. Much of this is compensation to business, communities and legal fees. Actual clean-up costs were only ~$14 billion and would be even less with NBS (The Wall Street Journal, 2016). |
Processing Algal Blooms in the Black Sea
Algae has numerous potential uses across a variety of industries that can contribute to sustainable development and environmental protection. There are thousands of different types of algae, though not all of them are directly useful for humans. However, many species of algae are known to be useful for a variety of applications.
Spirulina and Chlorella are protein, vitamin, and mineral-rich blue-green algae used in supplements and food. Kelp, a brown algae, is rich in iodine and minerals for dietary supplements and food production. Dunaliella produces beta-carotene for coloring and supplements. Haematococcus, a red algae, yields antioxidant astaxanthin for supplements and coloring. Diatoms, microalgae, serve industrial uses like filters and abrasives. Algae selection depends on intended purpose due to varying nutritional profiles and chemical compositions.
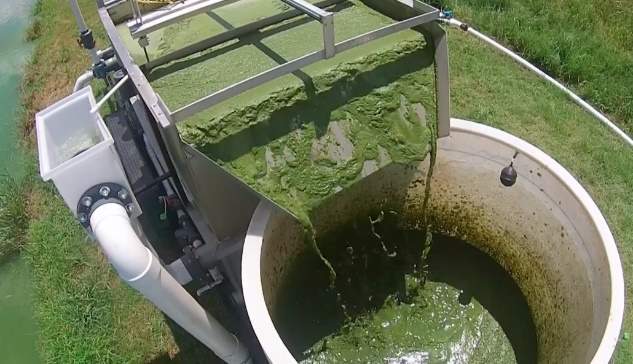
Algae can be harvested from the sea using various methods, including:
-
Mechanical Harvesting: This method involves physically collecting the algae using machines or equipment, such as trawls, dredges, or rakes. High concentration algae-water can be diverted with pumps and once accumulated, can be harvested once washed and dried.
-
Chemical Flocculation: This method involves adding chemicals, such as aluminum sulfate or ferric chloride, to the seawater to make the algae clump together and settle to the bottom. The resulting algae sludge is then collected and processed.
-
Bioreactors: This method involves growing algae in closed systems, such as tanks or tubes, with controlled environmental conditions, such as light, temperature, and nutrients. The harvested algae are then separated from the growth medium and processed.
-
Photo-bioreactors: This method is similar to bioreactors but utilizes light to grow algae more efficiently. Algae are grown on a large scale under optimized lighting conditions, and the harvested algae are separated from the growth medium.
Choice of harvesting method depends on several factors, such as the type of algae, the intended use, and the cost of the harvesting method. It is important to note that the harvesting process can have a significant impact on the environment and should be executed in an environmentally responsible manner. Once harvested, algae can have many potential uses, as listed below.
Table 5-9: Potential uses of algae
Potential uses of algae | Description of use |
---|---|
Food | Algae can be used as a source of protein and other nutrients in various food products, such as supplements, meat substitutes, and nutritional bars. |
Biofuels | Algae can be used as a feedstock for biofuels, such as biodiesel and bioethanol, because of their high lipid content. |
Agriculture | Algae can be used as a natural fertilizer and soil conditioner for crops. |
Pharmaceuticals | Algae contain a variety of bioactive compounds, such as antioxidants, anti-inflammatory agents, and anti-cancer agents, which can be used in pharmaceuticals and nutraceuticals. |
Cosmetics | Algae extracts can be used in cosmetic products for their moisturizing, anti-aging, and anti-inflammatory properties. |
Wastewater treatment | Algae can be used to treat wastewater by removing nutrients and other contaminants. |
Carbon capture | Algae can be used to capture and sequester carbon dioxide from industrial emissions. |
Bioplastics | Algae can be used as a feedstock for the production of bioplastics, which are biodegradable and have lower environmental impacts than traditional plastics. |
Aquaculture | Algae can be used as a source of food for aquaculture, such as fish and shrimp farming. |
A project in Türkiye is underway making use of algae to produce jet fuel and numerous other high-end products. If the collection of algae for further processing could be optimised this could be a potential win-win (EuronewsGreen, 2022).
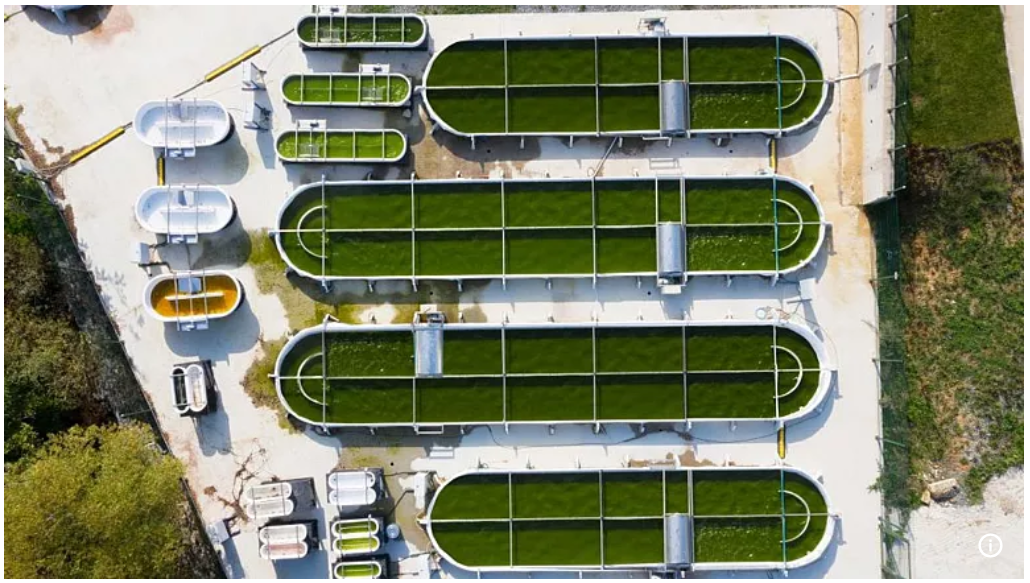
Table 5‑10: Project specifications for Bagazici University algae biorefinery
Indicator | Specification | Description |
Location | Place | Bogazici University, Istanbul, Türkiye |
Date | Project phase | 2020 |
Type of NBS | Algae removal and cultivation | |
Treatment type | Harvesting | |
Costs | Construction | €6 Million |
Operation (cost / year) | n.a. | |
Scale | Gross | ~ 2500m² |
Partners and financial mechanisms | Design | Race-ways, Processes 1200 t of wet algae mass / year |
Realisation | 85% financed by the EU, 15% Turkish Min. of Industry & Technology |
The Ocean Cleanup
The Ocean Cleanup's design philosophy, rooted in Nature-based Solutions, employs estuary interceptors and strategic positioning to address plastic pollution originating from rivers and coastal communities. The project has deployed estuary interceptors to catch debris prior to its entry into oceans, a critical measure given that 90% of debris, including plastic, originates from rivers. Learning from attempts to remediate plastic pollution the Great Pacific Garbage Patch, the most persistent debris is often a result of fishing industry practices and coastal communities. The systems strategically harness ocean currents, waves, and winds, positioned for optimal collection efficiency within gyres. The Black Sea's significant gyres and currents can be similarly harnessed for plastic collection. While effective for larger debris, such methods have limited efficacy against chemical or biological contaminants. Local initiatives like Most Valuable Catch have organized cleanup campaigns, retrieving kilometers of ghost netting with significant impact on marine life (HookandNetMagazine, 2021). However, these actions are temporary and not classified as enduring NBS solutions.
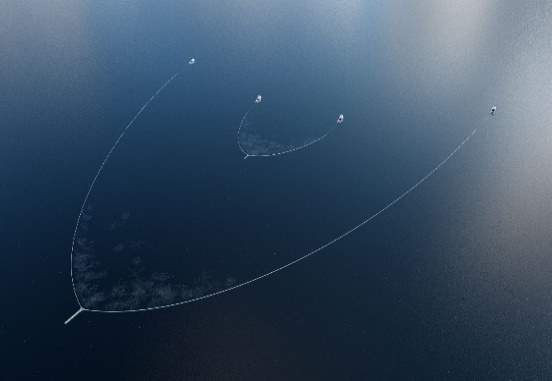
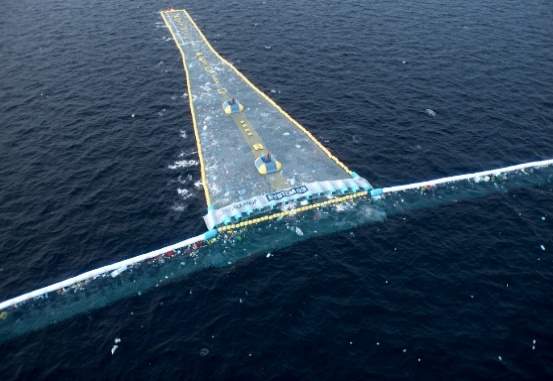
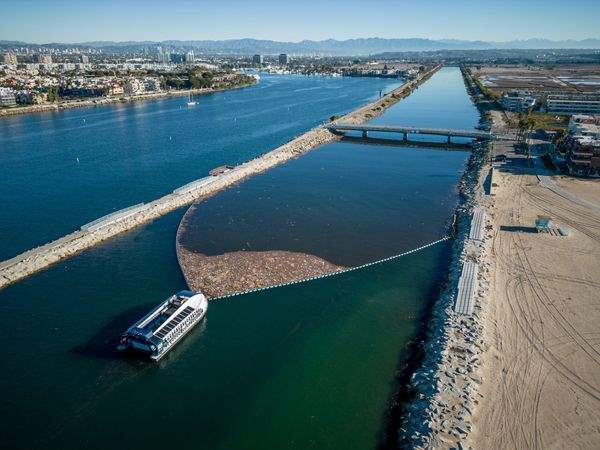
Figure 5‑11: Photos of Ocean Cleanup systems | Pictured: 002, 003 [which is 3 times longer than its predecessor], and interceptors
Table 5‑11: Project specifications for Ocean Cleanup operations in the Klang River (Malaysia)
Indicator | Specification | Description |
Location | Place | Klang River, Klang, Malaysia |
Date | Project phase | Since 2019, still in operation |
Type of NBS | n/a | |
Treatment type | Interceptor, physically collects and filters out plastic from river water | |
Costs | Construction | €252,000,000 (estimated for full coastline) |
Operation (cost / year) | n.a. | |
Scale | Gross | ̴ 8m x 24m x 5m |
Partners and financial mechanisms | Design | Barrier plus interceptor (conveyor belt processing ship), removes 100 t of rubbish/day |
Realisation | €700,000 donated by Cold Play |